HIGH-RESOLUTION X-RAY
ABSORPTION SPECTROMETRY OF ATOMIC VAPORS
Published
in:
A. Mihelič, A. Kodre, I. Arčon, and J. Padeľnik Gomilšek, Acta
Chim. Slov. (2004)
Abstract
A
measurement of an x-ray absorption spectrum of a monatomic sample yields
pure atomic absorption essential for the EXAFS (Extended X-ray Absorption
Fine Structure) analysis in cases of a weak structural signal. A construction
of an absorption cell to contain vapor sample takes into account specific
properties of the investigated element. Metal-vapor absorption cells
for the elements K, Zn, Rb, Cd, Cs, and Hg are presented and comments
are made on the use of materials and the operating mechanisms of the
cells.
Introduction
X-ray absorption
spectroscopy is a powerful spectroscopic technique that owes its popularity
among physicists and chemists mainly to its relative simplicity and,
especially in the last decades, to availability of synchrotron light
sources, where spectra with high energy resolution and signal to noise
ratio of the order 104 are attainable. In x-ray absorption spectra of
bound atoms, the absorption coefficient changes in an oscillatory fashion
with the energy of incident photons inside the region extending to about
1 keV above an absorption edge. These oscillations – the extended x-ray
absorption fine structure (EXAFS) – arise from scattering of the ejected
photoelectron on atoms surrounding the photoionised atom.1 EXAFS signal
contains information about the arrangement and atomic species of the
surrounding atoms and is used in structural analysis of materials. The
method is most useful for structural analysis of compounds without long-range
order where XRD (X-ray diffraction) methods cannot be used.
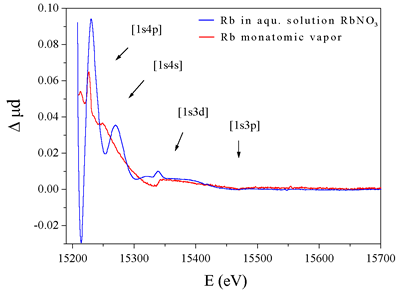 |
Fig.
1: The normalised K-shell X-ray absorption spectra
of atomic rubidium (top) and RbNO3 solution (bottom). |
The spectrum in
the energy region above an absorption edge contains, in addition to
EXAFS oscillations in the case of bound atoms, sharp spectral features
- fingerprints of collective motion of electrons inside the atom (Figure
1). These sharp features are produced by multielectron photoexcitations
(MPE), i.e., the processes where – beside the photoexcitation of the
inner shell of the atom – one or more additional electrons are ejected
to continuum or to unoccupied bound states.2 In materials with weak
EXAFS oscillations, the MPE and the EXAFS signals can be comparable
in magnitude, so that the EXAFS structural analysis can be seriously
hampered without prior knowledge of the MPE signal. Absorption spectra
of monatomic samples exhibit no EXAFS oscillations, whereas they do
contain the MPE signal. A straightforward way of obtaining an approximation
for the MPE in the spectra of bound atoms is therefore to measure the
absorption spectra in monatomic vapors of these elements. Readily amenable
to atomic measurements are noble gases, but with some experimental effort,
experiments with other elements which form monatomic vapors can also
be prepared. Apart from noble gases2-5, atomic absorption has been measured
only on some metals6-13, among which we measured for the first time
atomic absorption spectra of the most volatile metals Rb11, K12, Zn13,
Cs14, and Cd15, and remeasured Hg9. The experiments were performed at
three European beamlines: X1 and E4 of Hasylab, DESY (Rb/Cs and K, respectively),
and BM29 of ESRF, Grenoble (Zn, Cd and Hg).
For high quality x-ray absorption spectra a sample density of the order
of 10 mg/cm2 is required. Ionic and atomic beams, used in the visible
and the UV spectral regions, do not provide enough density and are therefore
inadequate. Dense metal vapors are contained in absorption cells with
low-absorption windows. Materials used for the cells and the windows,
which represent the most delicate part of the cell, must withstand the
aggressive vapor and the high temperatures needed to vaporise the metals.
The windows must be thin enough to transmit the incident beam sufficiently
and yet withstand some pressure difference. The investigated elements
differ in chemical reactivity and in the energy of their x-ray absorption
edges, i.e., their penetration; the choice of materials is therefore
specific to each of the elements.
Cadmium (BP 765 °C) and mercury (BP 357 °C) absorption spectra were
measured in simple sealed quartz cells. As the energies of Cd K edge
(26711 eV) and Hg L edges (14839, 14209, and 12284 eV) are rather high,
1 mm and 0.25 mm thick quartz windows, respectively, provided sufficient
transmission. Prior to sealing, the cells with the samples were introduced
and the cells were evacuated to 10-1 mbar. The Cd and Hg cells were
heated using a tubular oven to 950 °C and 340 °C, respectively, producing
vapor pressures of 168 kPa in the Cd cell and 28 kPa in the Hg cell.
The working temperature was chosen just high enough to evaporate the
sample entirely to assure that the vapor density remained constant throughout
the experiment.
Rb and Cs both combine with quartz at the boiling points of the both
elements (679 °C and 690 °C, respectively). For this reason, sealed
stainless steel cells11 with thin stainless-steel windows (0.01 mm)
were used (Figure 2). Vacuum tightness of the cells was tested with
a helium leak detector. The metals evaporated completely at 690 °C (Rb)
and 610 °C (Cs), yielding vapor pressures of 71 kPa and 34 kPa, respectively.
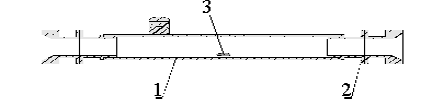 |
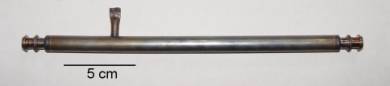 |
Fig.
2: Top: side view of the stainless-steel absorption
cell for rubidium and cesium vapor: 1 – tube, 2 – windows, 3 –
sample. Bottom: the cell after the experiment. |
Due to the low energy
of potassium K edge (3608 eV) and the consequently high absorption of
the x-ray beam in the windows, the only admissible materials for the
windows are organic foils, e.g., kapton. Organic materials have to be
protected from the hot and aggressive vapor (BP 774 °C). This is achieved
by the use of a spectroscopic heat-pipe12 depicted in Figure 3. A steady
circulation of vapor is established by heating the central part of the
stainless steel tube and water-cooling its outer parts. Shielding of
the windows is provided by a layer of an inert gas, coming from a reservoir
through gas inlets. The cell was filled with approximately 3 g of potassium.
A vapor pressure of 53 kPa was established at approximately 700 °C.
A two-hour stable operation rendered the absorption spectrum of the
vicinity of the potassium K edge.
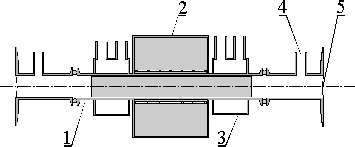 |
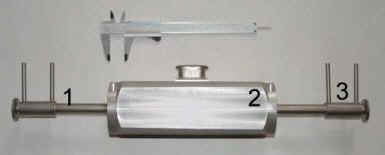 |
Fig.
3: Heat pipe cell for potassium vapor. Top: a schematic
view (1 – stainless steel tube, 2 – oven, 3 – water container,
4 – gas inlet, 5 – kapton windows). Bottom: the middle part of
the device; the ends with gas inlets are not shown. |
Zinc in vapor (BP
907 °C) is known to combine with quartz and stainless steel, the two
materials most commonly used in vacuum engineering, so we tried a corundum
cell13 with 0.125 mm corundum windows attached with a high-temperature
zirconia cement (Figure 4, top). The cell was not vacuum-tight; the
zinc vapors escaped slowly. Consequently, the batch of zinc exceeded
the quantity for the static (i.e., sealed-cell) filling to sustain a
stable vapor pressure. Inert atmosphere was provided to prevent oxidation
of the zinc vapor by inserting the corundum cell into a larger quartz
tube with aluminium windows. Baffles were inserted at both ends of the
tube to suppress convection and prevent zinc vapor to reach the aluminium
windows. The corundum cell and the quartz tube were heated to 750 °C
using the tubular oven; the absorption thickness of the sample corresponded
to a vapor pressure of approximately 21 kPa.
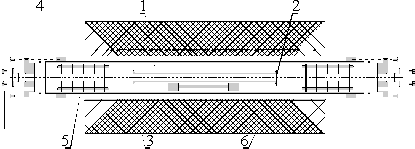 |
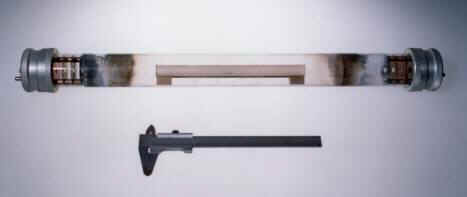 |
Fig.
4: Top: the absorption cell for zinc vapor: 1 – corundum
cell, 2 – alumina window, 3 – quartz tube, 4 – aluminium window,
5 – baffle, 6 – oven. Bottom: the cell after the experiment. |
References
1. J. J. Rehr, R. C. Albers, Rev. Mod. Phys. 2000, 72,
621-654.
2. S. J. Schaphorst, A. Kodre, J. Ruscheinski, B. Crasemann, T. Aberg,
J. Tulkki, M. H. Chen, Y. Azuma, G. S. Brown, Phys. Rev. A 1993, 47,
1953-1966.
3. J. M. Esteva, B. Gauthé, P. Dhez, R. C. Karnatak, J. Phys. B 1983,
16, L263-L268.