THE
ANTICANCER AGENT CISPLATIN ENCAPSULED INTO LIPOSOME MEMBRANES
Related
publication: I.
Arčon, A. Kodre, R.M. Abra, A. Huang, J. J. Vallner, D. D. Lasič Colloids
and Surfaces B: Biointerfaces, Vol. 33/3-4 (2004) 199-204
Introduction
Efficient and stable encapsulation of un-protonatable hydrophilic drugs
is one of the most difficult tasks in liposomal drug delivery [1].
In many cases, using high lipid and drug concentrations may be the
only way to achieve meaningful drug loading. However, formation of
homogeneous unilamellar vesicles in such conditions is often very
difficult or even impossible.
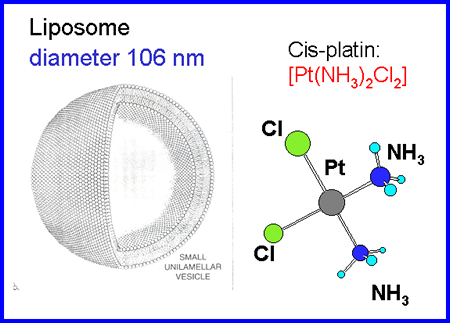 |
Fig.
1. Encapsulation
of anticancer agent cisplatin into liposomes |
The anticancer
agent cisplatin [2] is characterized by a solubility of approximately
1-2 mg/ml at room temperature, which increases to
8-10 mg/ml at 60°C; this solubility dependence on the temperature
can be exploited for efficient drug encapsulation. Liposomes can
be prepared
at high temperature, and on cooling the unencapsulated drug crystallizes
and can be separated (filtered or decanted) from drug?containing liposomes.
This procedure yields liposomes that contain in their aqueous core
cisplatin at approximately 8 mg/ml, i.e. about eight times its solubility
at room temperature, as shown by analytical methods and indirectly
by the anticancer activity of the formulation [3].
The drug-to-lipid ratio is ca. 0.01 mg/
M of total lipid. Cisplatin
does not leak out
from liposomes on storage and dilution with the external medium or
plasma, because of its insolubility in the bilayer and mechanically
very strong and impermeable bilayers.
Because the drug concentration exceeds its solubility eightfold, this
preparation raises the fundamental question of the physical state of
the drug in liposomes. The drug molecules can be precipitated, adsorbed
to the lipid surface, intercalated between polar heads, complexed with
(or dissolved within) polyoxyethylene chains grafted to the liposome
surface, or can simply form a supersaturated solution. Cryo electron
microscopy, IR (infrared), and Raman spectroscopy have not provided
any definitive answer to this question.
Experiment
Pt L3-edge EXAFS spectra were measured on the liposome-encapsulated
cisplatin to determine the local structure around Pt atoms in the
sample. For comparison, Pt L3-edge EXAFS of free drug in solid and
dissolved form was also measured, to give insight into the state
of the encapsulated drug.
Platinum L3-edge EXAFS spectra of the samples were measured in a transmission
mode at the X-ray beamline ROEMO2 (X1.1) in Hamburger Synchrotronstrahlungslabor
HASYLAB at Deutschen Elektronen-Synchrotron DESY (Hamburg, Germany). A Si(311)
fixed-exit double-crystal monochromator was used with 2-eV resolution at 12
keV. Harmonics were effectively eliminated by a slight detuning the monochromator
crystals using a stabilization feedback control. Ionization cells filled with
argon at 1 bar were used to detect the incident flux of the monochromatic x-ray
beam and the transmitted flux through the sample. Standard stepping progression
within a 1000-eV region above the edge was adopted with an integration time
of 1 s/point
Liquid liposome-encapsulated cisplatin samples and aqueous solution of cisplatin
were inserted in a variable-length liquid absorption cells with kapton windows.
Aqueous cisplatin solution (1 mg/ml) was prepared in situ by dissolving crystalline
cisplatin in distilled water. The optimal total absorption thickness (
d)
of about 2 was found with 5 mm thick sample layer in the cell in the case
of liquid
sample. Because of the very low concentration of Pt in the samples, the obtained
Pt L3 edge jump was only 0.04 and 0.1 for liposome-encapsulated sample and
the aqueous solution, respectively. Ten experimental runs were superimposed
in case of liposome-encapsulated samples and three runs in case of aqueous
solution to improve the signal-to-noise ratio. A reference spectrum for the
liposome-encapsulated samples was taken on a 5-mm thick layer of an aqueous
solution of placebo liposomes while reference spectrum for aqueous cisplatin
solution was measured on a 5-mm thick layer of distilled water. A powdered
crystalline sample was prepared on multiple layers of adhesive tape, with
Pt L3 edge jump of about 1. A reference spectrum was measured on empty tapes.
Results
EXAFS spectra were analyzed with the University of Washington analysis
program UWXAFS [9]. Standard k^2-weighted EXAFS spectra
(k) at
the Pt L3 edge for different cisplatin samples are shown in Fig.
2. Due
to low Pt concentration in the liposome-encapsulated sample, the
obtained signal-to-noise ratio is inferior to that in the crystalline
cisplatin and its aqueous solution.
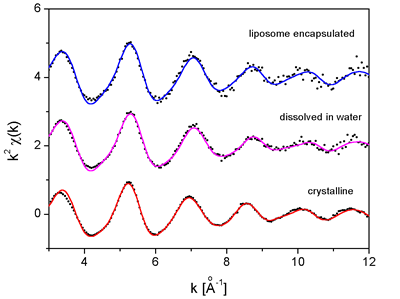 |
Fig.
2. k^2-weighted
Pt L3-edge EXAFS spectra of cisplatin in the crystal, in aqueous
solution, and in liposome-encapsulated
sample. Spectra are shifted vertically for clarity. Experiment
- dots; best fit model function - solid line. |
Fourier-transformed (FT) k^2-weighted EXAFS spectra calculated in
the k interval 3
to
12
for
individual samples are shown in Fig. 2. In the initial step,
the signal of the crystalline cisplatin
sample was analyzed. Although it was not directly relevant
for the study
of the encapsulation effects, this high-quality signal served
as a benchmark
of the achievable resolution. The model of the neighborhood was
built ad hoc with some help from crystallographic data [11,12].
Ref. 11
provides data on the immediate neighborhood of the Pt atom,
defined by the structure
of the molecule itself. Position of further neighbours is defined
by the particular stacking of the molecules in the crystal.
Two crystal
modifications of cisplatin are known. The sample used in this study
was identified by x-ray powder diffraction analysis as a monoclinic
"beta" form of Ref. 12.
Consequently, the R range of 1.0 .. 2.4
in
the FT spectrum was perfectly explained by a first-neighbor shell of
two N and two
Cl neighbors at
2.05 and 2.33 A, respectively. The further range between 2.4 and
4.0
was
resolved into contributions of higher-order scattering on the
first shell neighbors and of the second shell of neighbors, comprising
two Pt at 3.37
. The complete overview of best-fit parameters
is given in Table 1. The quality of the fit is shown on Figures
1 and
2.
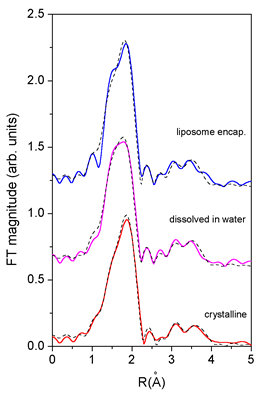 |
Fig.
3. The
k^2-weighted Fourier transform (k = 3 .. 12 ) of the EXAFS
spectrum of cisplatin in the crystal, in aqueous solution,
and
in liposome-encapsulated sample. Spectra are shifted vertically
for clarity. Experiment - solid line; best fit model function -
dashed line. |
The crystalline sample with its well-defined nearest neighbors
was also used to determine another parameter, the amplitude
reduction factor
of
the EXAFS signal for Pt atom. This number is transferable
between
different samples with the central atom in a similar chemical
(valence, coordination) state. The result (
= 0.77
0.03)
was in good agreement
with theoretical estimates [13] and
was used in the analysis of subsequent
cisplatin samples.
The EXAFS spectrum of the aqueous solution of cisplatin is another,
possibly closer template for identification of the Pt atom
neighborhood in the encapsulated samples. The model of the Pt neighborhood
was based on the closest shell of two N and two Cl atoms of
the
molecule,
as
in the crystalline sample. A very good fit (Table 1, Figs.
2 and 3) was obtained for the interval from 1.1 to 2.5
.
The structure parameters
are equal, within the error interval, to those of the crystalline
sample. With higher-order scattering contributions from the
same
shell the
validity of the model is extended up to 4
,
providing an explanation of the small double peak within the
region. The presence of
Pt atom neighbors in a second coordination shell, such as confirmed
in the
crystalline sample, was excluded on the basis of statistical
quality-of-fit
measure. EXAFS models that include Pt atoms in the second shell
do not fit the data in the R range from 2.5
to
4.0 
This was to be expected: the opposite finding would point to
aggregation of the cisplatin molecules in the solution, which,
if true, should
certainly have been supported by physicochemical data (osmotic
pressure, freezing-point depression). Diffuse vestiges of the
EXAFS signal
beyond 4
can be attributed to the hydration shell of the molecule.
Due to
random positions and motion of solvent molecules the signal
is largely averaged out. The important point, however, is that the
hydration
shell does not extend inward to the immediate vicinity of the
central Pt
atom.
The spectrum of the encapsulated cisplatin, although considerably
noisier, agree perfectly with that of the cisplatin solution.
The quantitative
analysis in the R interval from 1.1
to
2.5
confirms
the presence of two Cl and two N atoms in the first coordination
shell
at
the same distances as found in the solution, within the experimental
error (Table
1, Figs. 2 and 3). The presence of the second-shell Pt atom
neighbors is again excluded, so that aggregation of encapsulated cisplatin
molecules is not indicated.
Table 1. Parameters of the nearest coordination shells around
Pt in cisplatin samples: type of the neighbour atom, average number
N, distance R, and Debye-Waller factor s2. Uncertainty of the last
digit is given in parentheses. The r-factor is a measure of the
quality of EXAFS fit [9]. |
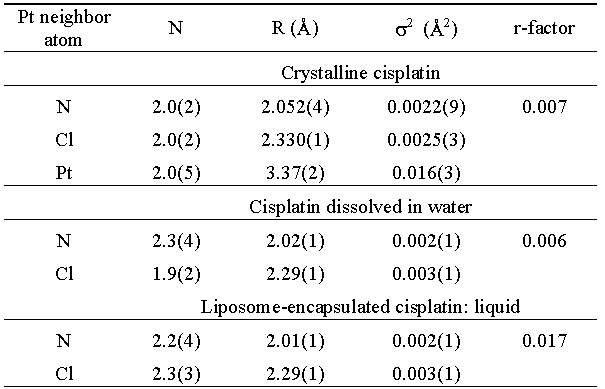 |
Discussion
The determination of the local structure in the neighborhood of the
Pt atom not only helps to determine the aggregation state but also
can shed light on its chemical structure and consequently, its chemical
stability. The first neighbors of the Pt atom, the directly bonded
Cl and N atoms, were found at the same distance and coordination
number in all samples, which shows that the cisplatin molecule in
the liposome is not appreciably affected by the physical state of
the system. This finding is supported by chemical and pharmacological
studies.
The observation of two N and two Cl atoms in the first coordination
shell demonstrates that the encapsulated cisplatin molecules are chemically
stable and do not hydrolyze, i.e. exchange one or two Cl atoms with
water. The absence of Pt neighbors at 3.37
clearly
demonstrates that the cisplatin solution inside the liposomes does
not crystallize. To
be more specific, the cisplatin solution does not crystallize in the
modification of the crystalline sample [11,12].
However, another crystal modification of cisplatin is known [14,15] -
a hydrated cisplatin in which the closest Pt neighbors are removed
to a distance beyond 4
,
on behalf of interposed water molecules that contribute two O neighbors
at the distance of 2.03
. Neither the encapsulated samples nor even
the plain solution show O atoms so close to the central Pt. Therefore,
the formation of hydrated crystal structures inside liposomes may be
excluded.
The data shown above, as well as NMR observations [5], suggest that
cisplatin in the liposome interior forms a supersaturated solution.
From the liposome size, and lipid and drug concentrations we can estimate
that each liposome contains on average approximately 2000 to 3000 cisplatin
molecules. It may be that the number of compartmentalized molecules
is simply too small to permit crossing the energy barrier from a crystal
embryo to a real crystal. If this is the case, the encapsulated cisplatin
molecules would constantly associate into crystallization nuclei and,
because there are not enough molecules to overcome the barrier to start
crystallization, constantly dissociate.
In conclusion, EXAFS data show that cisplatin forms a supersaturated
solution in liposomes in which the drug is chemically stable and does
not hydrolyze.
Acknowledgments
The study was supported by BMBF, Germany, and the Ministry of Sciences
and Technology, Slovenia. L. Troeger from HASYLAB provided expert
advice on beamline operation. We are thankful to A. Meden (University
of Ljubljana, Faculty of Chemistry and Chemical Technology) for the
powder x-ray diffraction analysis of the crystalline drug.