Large-pore
aluminophosphate molecular sieves
Related
publications:
1) I. Arčon,
N. Novak Tušar, A. Ristić, V. Kaučič, A. Kodre, M.Helliwell, J. Synch.
Radiation 8 (2001) 590-592
2)
A. Ristić, N. Novak
Tušar, I. Arčon, N. Zabukovec Logar, F. Thibault-Starzyk, J. Czyzniewska
and V. Kaučič, Chem.
Mater., 15 (2003) 3643-3649
3) Maja Mrak,
Nataša Novak Tušar, Alenka Ristič, Iztok Arčon, Frederic Thibault-Starzyk,
Venčeslav
Kaučič, Microporous and Mesoporous
Materials, 56 (2002) xxx
4) Alenka
Ristić, Nataša Novak Tušar, Iztok Arčon, Frederic Thibault-Starzyk, Darko
Hanžel, Jolanta
Czyzniewska and Venčeslav Kaučič, Microporous and Mesoporous
Materials, 56 (2002) 303
5) Iztok Arcon,
Nevenka Rajic, Alojz Kodre, J. Synch. Rad.
6 (1999) 460-461
Abstract
Large-pore metal modified aluminophosphate molecular sieves MeAPO-50
(Me=Co,Zn,Mn) were synthesised by hydrothermal crystallisation using
di-n-propylamine as structure directing agent. EXAFS and XRD analysis
show that cobalt(II), manganese(II) and zinc(II) are incorporated into
the non-phosphorus tetrahedral framework sites of MeAPO-50 on the threefold-axis
site, and on a site in a general position in the unit cell. Results
of both methods on distribution of the metal over the two sites are
compared.
Introduction
In recent years the interest in metal-substituted large-pore aluminophosphates
MeAPO-n (where Me = Co, Zn, Mn and n denotes a specific structure type)
molecular sieves has increased because of their use in catalytic reactions
with molecules of kinetic diameter greater than 0.7 nm (Davis,
1994).
The catalytic properties of these materials depend both on structure
and on the transition metal, i.e. its location and oxidation state.
Large-pore molecular sieve MeAPO-50 with a unidimensional 12-ring channel
system has a free aperture between 0.7 and 0.8 nm crossed-linked through
8 rings (Bennet, 1988). The unique feature of this structure type is
the high amount of metal cations incorporated into the framework. Metal
cations inhabit the MeAPO-50 framework on two nonequivalent non-phosphorus
tetrahedral sites. The first site (Me-1) on a general position with
multiplicity of 6 is preferably occupied by Al and by a small amount
of transition metal, while the second site (Me-2) on the threefold
axis with multiplicity 2 is occupied mainly by the substituting metal
cations.
In this paper we study the incorporation and distribution of Co, Zn,
and Mn cations into the MeAPO-50 molecular sieves by EXAFS and XRD
methods at the maximum amount of transition metal necessary for the
formation of the MeAPO-50 structure. In case of Mn, the distribution
of metal cations at the minimum amount of transition metal is analysed
for comparison.
Experiment
Large-pore CoAPO-50
and ZnAPO-50 products are synthesised by hydrothermal crystallisation
in the presence of di-n-propylamine as a structure
directing agent. The Me/Al ratio of 0.36 is used in the reaction
gel to obtain the product with the maximum amount of transition metal.
Details of the synthesis are described elsewhere (Ristić
et al., 1999).
MnAPO-50 is synthesised by the same procedure (Novak
Tušar et al., 2000) but with the Mn/Al ratio of 0.40 and 2.0 to obtain
the product
with the minimum (1-MnAPO-50) and the maximum amount of metal
(2-MnAPO-50), respectively.
Elemental analysis of the products was carried out by EDAX (Energy
dispersion analysis by X-ray) analytical system TRACOR
EDX, attached to the scanning electron microscope JXA-840A.
Single-crystal XRD analysis was performed on CoAPO-50 and
MnAPO-50 products, on Rigaku AFC5R rotating-anode diffractometer
using CuKa
radiation. The least-squares refinement of the hexagonal
unit cell parameters was performed on 23 reflections in
the range from 13.8 to
30.6 oq. TeXsan program package (Molecular
Structure Corporation, 1995) was used for all calculations. Data on ZnAPO-50 product
crystal structure
are obtained by Rietveld refinement of powder XRD patterns.
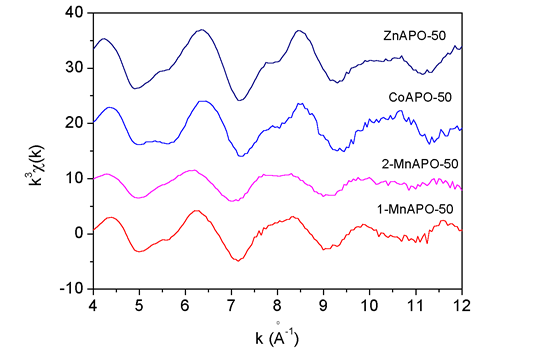 |
Fig.
1. The Zn, Co and Mn k3 EXAFS spectra measured
on ZnAPO-50, CoAPO-50, 1-MnAPO-50 and 2-MnAPO-50 samples. |
Powdered samples of the as-synthesized materials were prepared on multiple
layers of adhesive tape with the total absorption thickness of md
~2 above the K-edge. Their absorption spectra were measured at the
HASYLAB synchrotron facility at DESY (Hamburg, Germany). The E4 station
provides a focused beam from an Au-coated mirror and an Si(111) double-crystal
monochromator with 1.5 eV resolution at Mn K-edge. Harmonics were
effectively eliminated by a plane Au coated mirror and by a slight
detuning of the monochromator crystals using the beam stabilization
feedback control. The standard stepping progression within 1000 eV
region of K edge of the incorporated metal (Co, Mn, Zn) was adopted.
The monochromator scale was calibrated in energy by a simultaneous
absorption measurement on a thin foil of the respective metal. EXAFS
signal of the samples was obtained after removal of a reference spectrum
measured on empty tapes.
Results and discussion
The formation of a pure structural type MeAPO-50 is confirmed for
all samples by XRD analysis. The elemental analysis proves
the incorporation
of the metal cations into non-phosphorous sites, as evident also
from the stoichiometric formulae (Table 1). The occupancies
of each site
by metal cations obtained by XRD analysis are also shown. The data
for ZnAPO-50 are less reliable since they are obtained from powder
diffraction data.
Table
1. Stoichiometric
formulas from elemental analysis, percentage of metal cations
(p) on Me-1 and Me2 site, and fraction of metal (w1) on Me-1
site from XRD.
|
 |
The EXAFS spectra were analysed by UWXAFS and FEFF6 code (Stern
et al., 1995, Rehr et al., 1992) in the k-range 4 12 C-1, using k3 weight
and a Hanning window. The k3 weighted Co, Mn and Zn K-edge EXAFS spectra
of the samples are shown in Fig. 1.
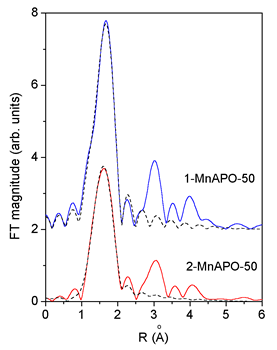 |
Fig.
2. Comparison of the k3 weighted FT magnitude of EXAFS spectra
measured on 1-MnAPO-50 and 2-MnAPO-50, (solid line) with the
FEFF model of the first peak (dashed
line). |
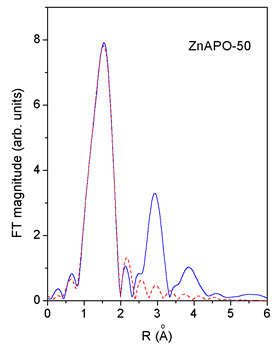 |
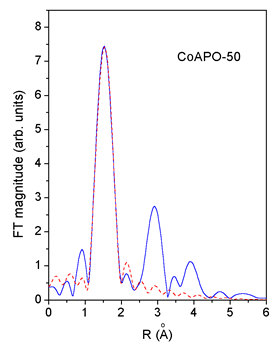 |
Fig.
3. Comparison of the k3 weighted FT magnitude of EXAFS spectra
measured on CoAPO-50 and ZnAPO-50 (solid line) with the FEFF
model of the first peak (dashed line). |
For a quantitative analysis a separate FEFF6 model of the local structure
around both sites (Me-1 and Me-2) is constructed, based on 1-MnAPO-50
crystallographic data (Novak Tušar et al., 2000). Mn2+ cation is tetrahedrally
coordinated by four oxygen atoms, but with significantly different
Mn-O distances 1.76 A and 2.04 A for Me-1 and Me-2 site respectively.
Thus, the analysis of the first coordination shell in the EXAFS spectra
in the R range of 1.0 C to 2.3 C is sufficient for determination of
distribution of the metal cation over both sites.
In modeling the EXAFS spectra, the scattering paths of the two
sites are combined. The ratio of the occupancies of the two
sites is varied
together with a common Debye-Waller factor s2 for the first coordination
shell. In addition, variable relative expansions DR/R for each site
are used to provide the relaxation of the Me-O distances from the
above Mn-O values obtained from MnAPO-50 diffraction data.
An excellent fit
is found for all the EXAFS spectra.The results are shown in Table
2. The quality of the fit is demonstrated on Figs. 2 and 3.
Table
2. Parameters
of the first coordination shell of metal cation on each metal
site in the crystal structure of MeAPO-50 samples: w - fraction
of metal at each
site. DR/R - lattice expansion relative to XRD 1-MnAPO-50 data (Novak Tušar,
2000) at each metal site, and s2 - Debye-Waller factor. Uncertainty of the
last digit is given in parentheses |
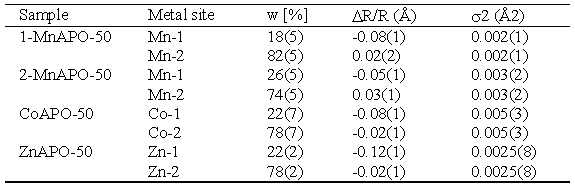 |
Co and Zn cations are incorporated into both crystallographic sites
Me-1 and Me-2 with significant predominance of the latter. The result
is similar for the minimum-Me 1-MnAPO-50 sample. In 2-MnAPO-50 sample
with the maximum amount of the metal the occupation of the Me-1 site
is increased.
These results of EXAFS analysis agree with X-ray diffraction structure
data of the products, where Me-2 site is found to be almost completely
populated with metal cations, while on the Me-1 site Al prevails.
This site accommodates Mn occupation between the lower and
upper limit of
MeAPO-50 structure viability.
However, precise quantitative agreement of the two methods is not
yet achieved.
Acknowledgement:
This work was supported by the Ministry of Science
and Technology of Slovenia and by Internationales Buero BMBF (Germany).
We are grateful to Dr. Marcus Tischer of HASYLAB who provided expert
advice on beamline operation.
References
Bennet, J.M. & Marcus, B.K. (1988). Innovations in Zeolite Material
Science, Studies in Surface Science and Catalysis, Vol. 37, edited
by Grobet, P.J., Mortier, W.J., Vansant, E.F. & Schitz-Ekloff,
G., pp. 269-279. Amsterdam: Elsevier.
Davis, M. E. (1994). Large pore molecular sieves, Catalysis Today 19,
1-212.
Molecular Structure Corporation, (1995). Single Crystal Structure Analysis
Software. Version 1.7. MSC. The Woodlands, USA.
Novak Tušar, N., Ristić, A., Meden, A. & Kaučič, V. (2000).
Microporous and Mesoporous Materials 37, 303-311.
Rehr, J.J., Albers, R.C. & Zabinsky, S. I. (1992). Phys. Rev
Lett. 69, 3397-3400.
Ristić,A., Novak Tušar, N., Zabukovec Logar, N., Mali,G., Meden,
A. & Kaučič,
V. (1999). Proceedings of the 12th International Zeolite Conference,
Vol. III, Materials Research Society, Warrendale, USA, 1585-1592.
Stern, E.A., Newville, M., Ravel, B., Yacoby, Y. & Haskel, D. (1995).
Physica B 208&209, 117-120.