EXAFS
DETERMINATION OF THE SIZE OF Co CLUSTERS ON SILICA
Related
publications:
1)
I.
Arčon, A. Tuel, A. Kodre, G. Martin, A. Barbier, J.
Synchrotron Rad., 8, (2001), p. 575-577 (reprint)
2) A.
Barbier, A.Tuel, I. Arčon, A. Kodre, G. Antonin Martin, Journal
of Catalysis Vol. 200 (2001) 106-116
Cluster size
The catalytic action
of metallic cobalt dispersed on microporous silica for the Fischer-Tropsch
reaction depends critically on the size of
the Co clusters. In the present study, an estimate of the average
cluster size in the samples prepared by three different routes is
deduced from the numbers of consecutive neighbours obtained in Co
K-edge EXAFS analysis.
Co/SiO2-1 sample was prepared by reaction of silica (silica FK 310,
800 m^2/g) with CoF3 in diglyme, to yield 1.83 wt % of Co in the
catalyst. Samples Co/SiO2-2 and Co/SiO2-3 were prepared by mixing silica
(A 200
from Degussa, 200 m^2/g) into aqueous solution of cobalt nitrate
with target Co concentrations in the catalyst of 17.6 wt % and 3.2
wt
%, respectively. With excess ammonia Co(OH)2 was precipitated. Finally,
all compounds were reduced under H2 at 923 K for 2 h.
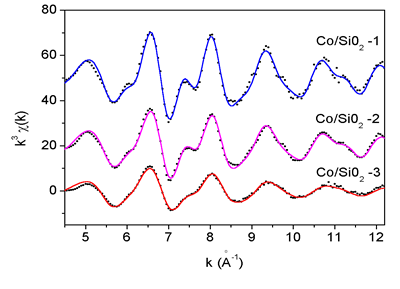 |
Fig.1. The
k^3 weighted Co K-edge EXAFS spectra of catalyst samples:
experiment
(dots) and best-fit (solid line). The spectra are
displaced vertically for clarity. |
EXAFS spectra at Co K-edge were measured at the E4 station of HASYLAB
at DESY. The station provides a focused beam from an Au-coated
mirror and a Si(111) double-crystal monochromator with 1.5
eV resolution at
Co K-edge. Harmonics are effectively eliminated by a plane Au coated
mirror and by a slight detuning of the monochromator crystals,
keeping the intensity at 60% of the rocking curve with the
beam stabilization
feedback control. Due to low concentration of Co in the samples,
the powder was compressed in a liquid absorption cell with
kapton windows
into a ~1 mm thick homogeneous layer, with Co K-edge jump of about
0.5 and total absorption thickness of 1.5 above the edge. Reference
spectra were measured on the empty absorption cell. EXAFS spectrum
of Co metal foil served as standard.
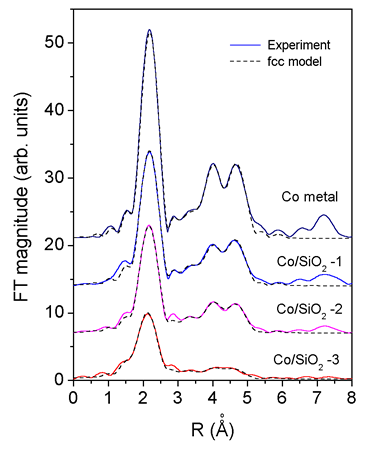 |
Fig.
2. The k^3 weighted Fourier transform magnitude
of Co K-edge EXAFS spectra of Co metal foil and catalysts calculated
in
the k
range of Fig. 1. The spectra
are displaced vertically for clarity. experiment
(solid line) and EXAFS model (dashed line). |
The EXAFS spectra
were analysed by the UWXAFS code [Stern
et al., 1995, Rehr et al., 1992] in the k-range 5 -12 A^(-1),
using k^3 weight and a Hanning window. The k^3 weighted Co K-edge
EXAFS spectra
of catalyst
samples Co-1, Co-2 and Co-3 are shown on Fig. 1. Fourier transforms
of the EXAFS spectra (Fig. 2) show typical local structure of metallic
Co in fcc crystal lattice in all samples. Comparison with the spectrum
measured on Co metal foil reveals, however, that the average number
of Co neighbours in the consecutive coordination shells in the
samples is lower than in the Co foil, decreasing from the sample
Co/SiO2-1
to Co/SiO2-3, indicating that clusters with fcc structure of Co
metal are formed. The size of the clusters can be deduced from the
observed
reduction of the average number of neighbours (Figure 3, 4) [Kakar
et al., 1997; Borowski, 1997; Frenkel, 1999]. For the Co-3 sample,
an estimate of the size can be given also by the cutoff in the
FT spectrum. The absence of a prominent peak observed at 7.5 A in
FT
spectrum of bulk Co provides an upper limit for the diameter.
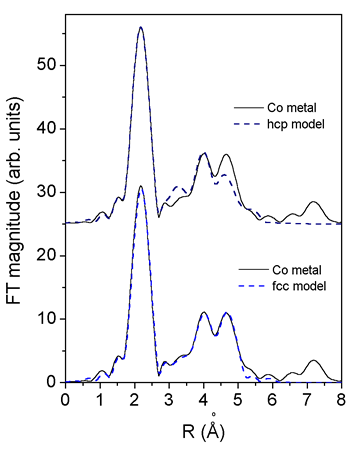 |
Fig.
3. Comparison
of Fourier transform of the EXAFS spectrum of Co metal foil
with EXAFS models for fcc and hcp crystal structure. experiment
(solid line) and EXAFS model (dashed line). Metallic
Co foil shows
typical local structure of fcc crystal lattice, hcp model cannot
satisfactorly describe measured spectrum in the R range above
3 A.
|
A quantitative analysis is based on FEFF model of fcc crystal structure
of Co metal with the lattice constant a = 3.61 A, comprising all
single and multiple scattering paths up to 5.9 A. The model
is calibrated
by the Co foil spectrum, yielding an excellent fit for the region
from 1.5 C to 5.0 A with just four variable parameters: the
lattice expansion
,
the amplitude reduction factor
,
the Debye temperature (TD) in modeling the Debye-Waller factors
of all paths (Stern et
al., 1995), except the first, for which a separate factor
is introduced.
The shell coordination numbers and unperturbed radii are fixed at
their fcc values.
In modeling the clusters, the fixed values of
(=0.86(3))
is retained, while TD,
and
are varied, and the fcc shell coordination numbers in the model
are multiplied with variable average neighbour
fractions
(ni) with the results in Table I. The quality of the fit is demonstrated
in Figs. 1 and 2.
For Co/SiO2-3 sample an additional shell of 0.8(3) oxygen atoms at
R= 1.97(3) A
with
= 0.004(1) A^2 has to be introduced
for a fit of equivalent quality.
Table
1. Average neighbour fractions (ni) of Co neighbours
in the first four coordination shells in the Co samples. Best
fit values for the lattice expansion , the Debye-Waller
factor of the first coordination shell s12 and the Debye temperature
(TD) governing the widths of further shells are added. Uncertainties
of the last digit are given in parentheses. |
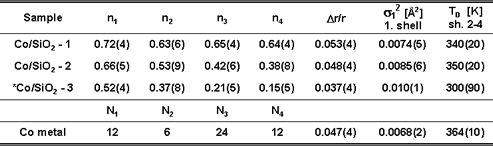 |
The average neighbour fractions (ni) of the first four shells in
the Co/SiO2-1, Co/SiO2-2, and Co/SiO2 -3 samples are compared
with values
from model fcc magic-number clusters. These clusters are obtained
by filling consecutive crystallographic shells, i.e. shells of
sites with
all permutations of the same crystallographic indices with regard
to the central atom. The model neighbour fractions are obtained
by summing
the number of i-th neighbours over all atoms of the cluster and
dividing by the corresponding number in the bulk. (Table 2).
The best agreement
(bold rows) is obtained with clusters containing 135, 55 and
19 atoms (diameters of 13.5, 10.2, and 7.2 A) for Co/SiO2-1,
Co/SiO2-2,
and
Co/SiO2-3, respectively. The agreement in all four shells for
the two smaller clusters is remarkable. It is possible that
the larger
deviations
for Co-1 clusters indicate deformation from the globular shape.
Table
2. Model: average neighbour fraction (ni) of consecutive
neighbours in fcc magic-number clusters. |
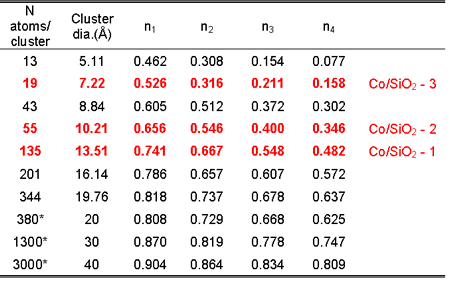 |
The oxygen neighbours observed in Co/SiO2-3 sample presumably
originate from the contact layer with the substrate. Their
contribution is
appreciable only for the smallest clusters where most of the
Co atoms in the cluster
are on its surface.
An increase of the Debye-Waller factors (and a corresponding
decrease of Debye temperature) from the bulk Co value is
observed in all
three cluster samples. The effect is more pronounced for
smaller clusters
and can be ascribed to the increase in the static disorder
in the clusters [Kakar et al., 1997;
D'Acapito et al., 1997].
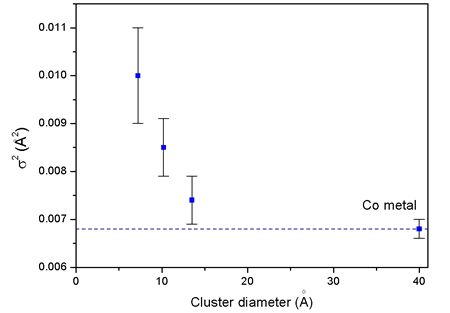 |
Fig.
4. Debye-Waller
factors of the first coordination shell around Co in clusters of
different size compared to the bulk Co value (dashed line). |
We observe a reduction of interatomic distances only for
the smallest cluster size (Co/SiO2-3 sample), consistent
with similar
observations
for Cu clusters [Montano et al., 1986; D'Acapito et al.,
1997). Larger clusters of Co/SiO2-1 and Co/SiO2-2 samples
show no
significant contraction
of the interatomic distances from their bulk Co values.
Acknowledgment: M.
Tischer from HASYLAB provided expert advice on beamline operation.
The study is supported by Internationales Bhro des BMBF, Germany,
and Ministry of Sciences and Technology, Slovenia.