Mezoporous
catalytical molecular sieves: Silicalite-1
Related
publications:
1) N. Novak Tušar,
N. Zabukovec Logar, I. Arčon, F. Thibault-Starzyk, A. Ristić, N. Rajić,
Venčeslav
Kaučič, Chemistry of Materials 15 (2003)
4745-4750
2)
N. Novak Tušar,
N. Zabukovec Logar, I. Arčon, F. Thiboult-Starzky, V. Kaučič, Croatica
Chemica Acta, 74 (2001) 837-849
3) A. Barbier,
A.Tuel, I. Arčon, A. Kodre, G. Antonin Martin, Journal of Catalysis
Vol. 200 (2001) 106-116
Abstract
Mn- and Co-containing
silicalite-1 (MnS-1 and CoS-1) were synthesized hydrothermally. Template-free
samples were obtained by calcination
at 550°C for 6 hours in oxygen flow. X-ray diffraction, elemental,
thermogravimetric and cation exchange analyses suggested a possible
in-corporation of Mn and Co cations into the framework positions of
the silicalite-1. The valence state and local environment of both cations
in the samples were examined by XANES and EXAFS analyses. Results show
that Mn and Co cations isomorphously substitute silicon in the silicalite-1
framework only if they are in trivalent oxidation state. Divalent cations
are incorpo-rated on extra-framework positions. In the template-free
MnS-1 sample all Mn cations are in tri-valent state and substitute
Si in the framework, forming a distorted and coordinatively unsatu-rated
3-fold symmetry, which is characteristic for Lewis acid sites. In the
template-free CoS-1 sample only part of Co cations are in trivalent
state substituting silicon in the framework.
Introduction
Zeolites with aluminosilicate framework are crystalline oxides comprising
corner-sharing TO4 tetrahedra (T=Si, Al) [1]. They possess regular
pore system with diameters in the range from 0.3-1.4 nm and act as
sieves at molecular level.
Silicalite-1 is an aluminium-free zeolite with the MFI structural
topology [1]. Modification of silicalite-1 by isomorphous substitution
of silicon
by transition-metal elements enhances its catalytic activity. Incorporation
of tetravalent (e.g. Ti, V) transition metals into the framework
of silicalite-1 results in novel molecular sieves with selective
oxidation
properties [2]. Incorporation of trivalent (e.g. Cr, Fe) transition
metals into the framework of silicalite-1 results in high-quality
inorganic membranes used for catalytic membrane reactors [3].
So far all attempts
of incorpora-tion of divalent transition metals (e.g. Mn, Co) lead
to the formation of the silicalite-1 with diva-lent cations on extra-framework
positions [4].
In this paper we report on the isomorphous substitution of silicon
in the framework of sili-calite-1 by manganese and cobalt cations.
Mn and Co K-edge XANES analysis is used to probe directly the valence
state of the transition metal cations in the as-synthesized and template-free
samples, while their local environment and therewith the site of
the incorporation is examined by EXAFS.
Experimental
Manganese-containing silicalite-1 (MnS-1) was syntesized hydrothermally
using tetraethyl-ammonium hydroxide (TEAOH) as a structure-directing
agent (template) [5]. Cobalt-containing silicalite-1
(CoS-1) was synthesized by similar procedure using tetrapropylammonium
hydroxide
(TPAOH) as a template. Template-free products were prepared by calcination
at 550°C for 6 hours in oxygen flow. Cation exchange was performed
at room temperature by stirring the solid sample in a 1M solution
of NaCl for 24 hours with solid/liquid mass ratio 1/10. X-ray powder
diffraction (XRPD) patterns of the products were collected on a Siemens
D-5000 diffractometer with CuKa radiation. Thermogravimetric analysis
(TGA) was performed on a TA 2000 thermal analyzer in static air.
Elemental analysis was carried out using an EDXS (energy dispersive
X-ray spectroscopy) anaysis within the LINK ISIS 300 system, attached
to the scanning electron microscope JEOL JSM 5800.
X-ray absorption spectra of MnS-1, CoS-1 and reference samples
in the respective energy regions of the Mn and Co K-edge were
measured at
E4 beamline of HASYLAB synchrotron fa-cility at DESY in Hamburg.
The beamline provided a focused beam from an Au-coated torroidal
mirror
and a Si(111) double crystal monochromator with about 1 eV resolution
at 7 keV. Har-monics were effectively eliminated by a plane Au-coated
mirror, and by a slight detuning of the monochromator crystals, keeping
the intensity at 60% of the rocking curve with the beam stabili-zation
feedback control. Powder samples were prepared on multiple layers
of adhesive tape. Sev-eral layers were stacked to obtain optimal
attenuation
above the K-edge of the investigated ele-ment. Reference spectra
were measured under the same conditions on empty tapes without
the sample.
The standard stepping progression within [-250 eV .. 1000 eV] region
of the Mn and Co K-edge was adopted for EXAFS spectra with an integration
time of 4 s/step. Exact energy cali-bration was established with
the simultaneous absorption measurements on Mn and Co metal.
Results and discussion
The as-synthesized MnS-1 and CoS-1 were identified from XRPD patterns
as single crystalline phase products with MFI structure. Elemental
and thermogravimetric analyses of the as-synthesized and template-free
MnS-1 and CoS-1 suggested that 0.5 % Si was isomorphously sub-stituted
by Mn and Co, respectively. Cation exchange procedure revealed that
there were no ex-changeable cationic sites in the template-free MnS-1,
while some exchangeable cationic sites were present in the template-free
CoS-1 sample. This indicated that all incorporated manganese atoms
in the template-free sample isomorphously substituted framework silicon
in the silicalite-1, while the Co substitution in the template-free
CoS-1 sample sample was only partial.
To determine the valence state of the transition metal cations
in the as-synthesized and cal-cined samples we examined Co
and Mn K-edge
energy
shifts in the absorption spectra of the samples. The precise energy
position of the edge was taken at the edge inflection point. A
linear relation between the edge shift and the oxidation state
was established
for the atoms with the same type of ligands [6-9]. For manganese
atoms coordinated to oxygen atoms a shift of 3.5 eV per oxidation
state was
found [7], while for cobalt atoms shifts of 1.5 - 3 eV per valence
were reported [9].
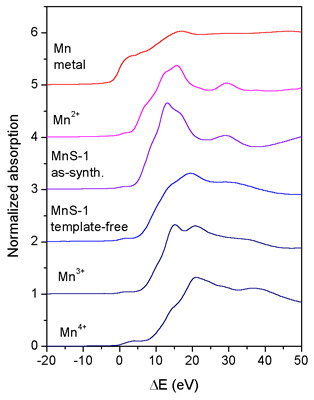 |
Fig.
1. Normalized Mn K-edge XANES spectra of the as-synthesized
and template-free MnS-1 and Mn reference samples Mn metal,
Mn2+O, K3[Mn3+(C2O4)3].3H2O, and Mn4+O2. The spectra are displaced
vertically for clarity. The zero energy is taken at the first
inflection point of the Mn K-edge in the spectrum of Mn metal
(6539.0 eV). |
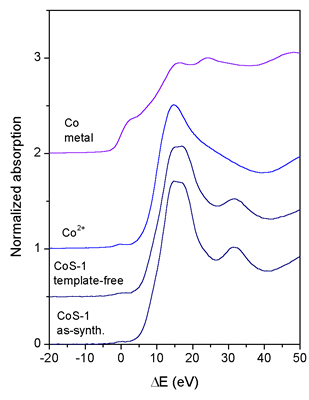 |
Fig.
2. Normalized Co K-edge XANES spectra of the as-synthesized
and template-free CoS-1 and Co reference samples Co metal and
Co2+(CH3COO)2ˇ4H2O. The spectra are displaced vertically for
clarity. The zero energy is taken at the first inflection point
of the Co K-edge in the spectrum of Co metal (7709.0 eV). |
In Fig. 1 the Mn XANES spectra of the as-synthesized and template-free
MnS-1 sample to-gether with the spectra of the reference manganese
compounds Mn2+O, K3[Mn3+(C2O4)3].3H2O, and Mn4+O2 with known oxidation
states are shown. The edge shift in the as-synthesized MnS-1 is
the same as in the Mn2+O compound, indicating that the average
oxidation
state of manganese in the as-synthesized MnS-1 is 2+. The Mn K-edge
in the template-free MnS-1 sample is shifted and coincides with
the edge shift in the K3[Mn3+(C2O4)3].3H2O compound. We can
thus conclude
that during calcination all Mn+2 cations oxidize to Mn+3.
The Co XANES spectra (Fig. 2) reveal completely different behaviour
of Co cations in the CoS-1 samples. The same energy position
of Co K-edge is found in both as-synthesized and tem-plate-free
CoS-1
samples,
demonstrating that the valence state of the incorporated Co cations
does not change during the process of calcination. Furthermore,
the Co K-edge in the CoS-1 samples is shifted for about 1 eV
relative to the edge in the reference Co2+ acetate sample,
which indicates
the
presence of Co2+ and Co3+ cations in the silicalite samples.
Mn and Co K-edge EXAFS spectra of the as-synthesized and template-free
MnS-1 and CoS-1 samples were quantitatively analyzed for the
coordination number, distance, and Debye-Waller factor of the
nearest coordination
shells of neighbor atoms using the University of Wash-ington
UWXAFS package [10] and FEFF6 code [11]. The amplitude reduction
factor
(So2 = 0.80) is kept fixed in the fits of all spectra. Its value
is obtained
from previous experimental results [12]. Fourier transforms of
Mn and Co EXAFS spectra are shown in Figs. 3 and 4 together with
best-fit
EXAFS models. Complete lists of best-fit parameters are given
in Tables
I and II.
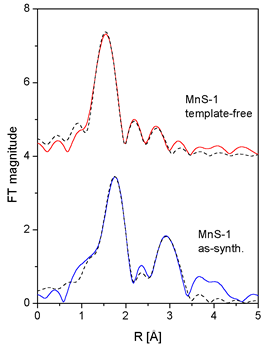 |
Fig.
3. Fourier transforms of the k3-weighted Mn EXAFS
spectra of the as-synthesized and template-free MnS-1 sample,
calculated in the k range of 5-12 A-1 (solid line - ex-periment,
dotted line - EXAFS model). |
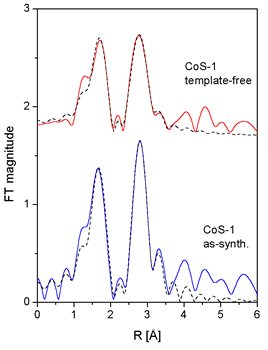 |
Fig.
4. Fourier transforms of the k2-weighted Co EXAFS
spectra of the as-synthesized and template-free CoS-1 sample,
calculated in the k range of 3.5-12 A-1 (solid line - ex-periment,
dotted line - EXAFS model). |
Two shells of neighbours are found around Mn cations in the as-synthesized
MnS-1 sam-ple. The fit in the R range from 1.2 A to 3.5 A
shows that the first shell is composed of four oxy-gen atoms
at 2.18
A, while
the second shell comprises about two Mn atoms at 3.35 A.
The pres-ence of Mn-O-Mn links indicates that Mn cations (divalent
as shown by
XANES) are located at extraframework sites, most probably
in
the form of metal-oxo
species coordinated to the frame-work, but simultaneously
bearing extra-framework oxygen atoms [7].
Significantly different local Mn neighborhood (within the
same R range) is found in the template-free
MnS-1 sample. Mn is
coordinated to three
oxygen atoms in the first coordination
shell, two of them at a shorter
distance of 1.93 A and one at a
longer distance of 2.15 A. The short distance of 1.93 A is
consistent with the average
tetrahedral
Mn+3-O
distance of 1.93(4) A reported
for MnAsO4 [13]. In the second coordination shell two oxygen
atoms were identified at 2.81
A and 3.04 A. Additionally,
at a larger distance of about 3.5
A a presence of silicon atoms is indicated. Taking into account
XANES results we
can conclude
that
Mn3+ cations substitute Si in the
framework of the template-free MnS-1,
forming a distorted and coordination-wise
unsatu-rated 3-fold symmetry, which is characteristic for Lewis
acid sites (manganese
framework
sites) [14].
In modeling Co EXAFS spectra, the
fit in the R range 1.3 A 3.5
A shows that in the as-synthesized
sample cobalt
atoms
are
coordinated
to four oxygen atoms at 2.10
A, while the sec-ond shell is composed
of Co and O atoms. The local
Co neighborhood in
the template-free
sam-ple
is slightly different (Fig. 4,
Table II) but the Co-O-Co links
are retained. Combining Co cation
exchange, XANES and
EXAFS
results, we may conclude that
the template-free CoS-1 sample contains
two Co species
(Co2+ and Co3+), where trivalent
cobalt cations substitute
silicon in the framework sites
while divalent Co cations are
located at extra-framework
sites in the form of metal-oxo
species.
In conclusion, the results show
that Mn and Co cations substitute
silicon in the silicalite-1 framework
isomorphously only if they are
in the
trivalent oxidation state. Divalent
cations incor-porate into the
silicalite-1 on the extra-framework
positions.
Acknowledgment
We acknowledge the support by the Slovenian Ministry of Education,
Science and Sport through the research program P0-0516-0104 and the
project Z2-3457-0104, by Internationales Buero BMBF (Germany), and
by the IHP-Contract HPRI-CT-1999-00040 of the European Commis-sion.
Advice on beamline operation by Konstantin Klementiev of HASYLAB
is gratefully ac-knowledged.
Table
1. Structural parameters of the nearest coordination
shells around Mn atom in as-synthesized and template-free
MnS-1: type of neighbor atom, average number N, distance
R, and Debye-Waller factor s2. Uncertainties in the last
digit (estimated by UWXAFS fitting program) are given in
the parentheses.
|
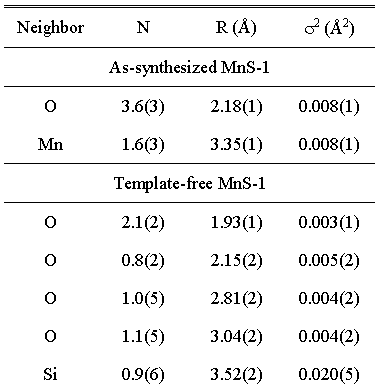 |
Table
2. Structural parameters of the nearest coordination
shells around Co atom in as-synthesized and template-free
CoS-1: type of neighbor atom, average number N, distance
R, and Debye-Waller factor s2. Uncertainties in the last
digit (estimated by UWXAFS fitting program) are given in
the parentheses.
|
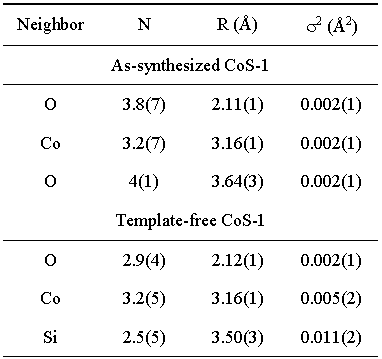 |
References
[ 1 ] Depmeier, W., Handbook of Porous Solids. (Edited by F. Schüth,
K.S.W. Sing, J. Weit-kamp) (Wiley-VCH, Weinheim 2002), vol. II, p.
728.
[ 2 ] Mal, N.K. and Ramaswamy, P., Appl. Catal. A: General 143, 75
(1996).
[ 3 ] Li, S., Tuan, V.A., Falconer, J.L. and Noble, R.D., Micropor.
Mesopor. Mater. 58, 137
(2003).
[ 4 ] Wichtelová, B., Sobalík, Z. and Dědeček, J., Appl. Catal. B:
Environmental 41, 97 (2003).
[ 5 ] Tušar, N.N. et al., Chem. Mater., sent for publication.
[ 6 ] Wong, J., Lytle, F.W., Messmer, R.P. and Maylotte, D.H., Phys.
Rev. B 30, 5596 (1984).
[ 7 ] Ressler, T., Brock, S.L., Wong, J. and Suib, S.L., J. Synchrotron
Rad. 6, 728 (1999).
[ 8 ] Arčon, I., Mirtič, B. and Kodre, A., J. Am. Ceram. Soc. 81, 222
(1998).
[ 9 ] Barrett, P.A., Sankar, G., Catlow, C.R.A. and Thomas, J.M., J.
Phys. Chem. 100, 8977
(1996).
[10] Stern, E.A., Newville, M., Ravel, B., Yacoby, Y. and Haskel, D.,
Physica B 208-209, 117 (1995).
[11] Rehr, J.J., Albers, R.C. and Zabinsky, S.I., Phys. Rev. Lett.
69, 3397 (1992).
[12] Tušar, N.N. et al., Microporous and Mesoporous Mat. 55, 203 (2002).
[13] Aranda, M.A.G., Attfield, J.-P. and Bruque, S., Inor. Chem. 32,
1925 (1993).
[14] Zibrowius, B. and Loffler, E. Handbook of Porous Solids. (Edited
by F. Schüth, K.S.W.
Sing, J. Weitkamp) (Wiley-VCH, Weinheim 2002), vol. II, p. 983.
|
|
|
|
 |
|